How Light Behaves
During traveling light waves interact with matter. The consequences of this interaction are that the waves are scattered or absorbed. In the following, we describe the principal behaviors of light.
SCATTERING
Scattering is the process by which small particles suspended in a medium of a different density diffuse a portion of the incident radiation in all directions. In scattering, no energy transformation results, there is only a change in the spatial distribution of the radiation (Figure 5.2).
In the case of solar radiation, scattering is due to its interaction with gas molecules and suspended particles found in the atmosphere. Scattering reduces the amount of incoming radiation reaching the Earth’s surface because significant proportion of solar radiation is redirected back to space.
Some molecules have the ability to absorb incoming light. Absorption is defined as a process in which light is retained by a molecule. In this way, the free energy of the photon absorbed by the molecule can be used to carry out work, emitted as fluorescence or dissipated as heat.

FIGURE 5.3 Light absorption by a unicellular alga: II, light incident on the cell and IT, light transmitted by the cell

FIGURE 5.3 Light absorption by a unicellular alga: II, light incident on the cell and IT, light transmitted by the cell
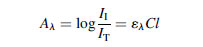
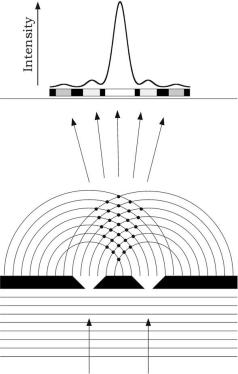
FIGURE 5.4 Interference of light passing through two narrow slits, each acting as a source of waves. The superimposition of waves produces a pattern of alternating bright and dark bands. When crest meets crest or
trough meets trough, constructive interference occurs, which makes bright bands; when crest meets trough destructive interference occurs, which makes dark bands. The dots indicate the points of constructive interference. The light intensity distribution shows a maximum that corresponds to the highest number of dots.
Electromagnetic waves can superimpose. Scattered waves, which usually have the same frequency, are particularly susceptible to the phenomenon of interference, in which waves can add constructively or destructively. When two waves, vibrating in the same plane, meet and the crests of one wave coincide, with the crests of the other wave, that is, they are in phase, then constructive interference occurs. Therefore, the amplitude of the wave has been increased and this results in the light appearing brighter. If the two waves are out of phase, that is, if the crests of one wave encounter the troughs of the other, then destructive interference occurs. The two waves cancel out each other, resulting in a dark area (Figure 5.4). The interference of scattered waves gives rise to reflection, refraction, diffusion, and diffraction phenomena.
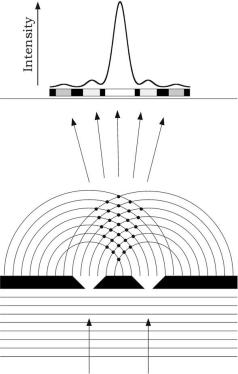
FIGURE 5.4 Interference of light passing through two narrow slits, each acting as a source of waves. The superimposition of waves produces a pattern of alternating bright and dark bands. When crest meets crest or
trough meets trough, constructive interference occurs, which makes bright bands; when crest meets trough destructive interference occurs, which makes dark bands. The dots indicate the points of constructive interference. The light intensity distribution shows a maximum that corresponds to the highest number of dots.