Subphylum Crustacea
Subphylum
Crustacea
General Nature of a Crustace
Crustaceans differ from other arthropods in a variety of ways, but the only truly distinguishing characteristic is that crustaceans are the only arthropods with two pairs of antennae. In addition to two pairs of antennae and a pair of mandibles, crustaceans have two pairs of maxillae on the head, followed by a pair of appendages on each body segment or somite. In some crustaceans not all somites bear appendages. All appendages, except perhaps the first antennae, are primitively biramous (two main branches), and at least some appendages of present-day adults show that condition. Organs specialized for respiration, if present, function as gills.
Most crustaceans have between 16 and 20 somites, but some forms have 60 somites or more. A larger number of somites is a primitive feature. The more derived condition is to have fewer segments and increased tagmatization. Major tagmata are head, thorax, and abdomen, but these are not homologous throughout the class (or even within some subclasses) because of varying degrees of fusion of somites, for example, as in the cephalothorax.
By far the largest group of crustaceans is class Malacostraca, which includes lobsters, crabs, shrimps, beach hoppers, sow bugs, and many others. These show a surprisingly constant arrangement of body segments and tagmata, which is considered the ancestral plan of the class (Figure 19-1). This typical body plan has a head of five (six embryonically) fused somites, a thorax of eight somites, and an abdomen of six somites (seven in a few species). At the anterior end is a nonsegmented rostrum and at the posterior end is a nonsegmented telson, which with the last abdominal somite and its uropods forms a tail fan in many forms.
In many crustaceans the dorsal cuticle of the head may extend posteriorly and around the sides of the animal to cover or be fused with some or all of the thoracic and abdominal somites. This covering is called a carapace. In some groups the carapace forms clamshell-like valves that cover most or all of the body. In decapods (including lobsters, shrimp, crabs, and others), the carapace covers the entire cephalothorax but not the abdomen.
Form and Function
Because of their size and easy availability, large crustaceans such as crayfishes have been studied more than other groups. They are also commonly studied in introductory laboratory courses. Therefore many of the comments that follow apply specifically to crayfishes and their relatives.
External Features
The bodies of crustaceans are covered with a secreted cuticle composed of chitin, protein, and calcareous material. The harder, heavy plates of larger crustaceans are particularly high in calcareous deposits. The hard protective covering is soft and thin at the joints between somites, allowing flexibility of movement. The carapace, if present, covers much or all of the cephalothorax; in decapods such as crayfishes, all head and thoracic segments are enclosed dorsally by the carapace. Each somite not enclosed by the carapace is covered by a dorsal cuticular plate, or tergum (Figure 19-2A), and a ventral transverse bar, the sternum, lies between the segmental appendages (Figure 19-2B). The abdomen terminates in a telson, which is not considered a somite and bears the anus. (The telson may be homologous to the annelid pygidium.)
The position of the gonopores varies according to sex and group of
crustaceans. They may be on or at the
base of a pair of appendages, at the
terminal end of the body, or on
somites without legs. In crayfishes the
openings of the vasa deferentia are on
the median side at the base of the fifth
pair of walking legs, and those of the
oviducts are at the base of the third
pair. In females the opening to the
seminal receptacle is usually located in
the midventral line between the fourth
and fifth pairs of walking legs.
Appendages: Members of classes Malacostraca (including crayfishes) and Remipedia typically have a pair of jointed appendages on each somite (Figure 19-3), although the abdominal somites in the other classes do not bear appendages. Considerable specialization is evident in appendages of derived crustaceans such as crayfishes. However, all are variations of the basic, biramous plan, illustrated by a crayfish appendage such as a maxilliped (a thoracic limb modified to become a head appendage) (Figures 19-3 and 19-4). The basal portion, or protopod, bears a lateral exopod and a medial endopod. The protopod is made up of one or two joints (basis and coxa), whereas the exopod and endopod have from one to several joints each. Some appendages, such as walking legs of crayfishes, have be-come secondarily uniramous. Medial or lateral processes sometimes occur on crustacean limbs, called endites and exites, respectively, and an exite on the protopod is called an epipod. Epipods are often modified as gills. Table 19-1 shows how the various appendages have become modified from the biramous plan to fit specific functions.
Structures that have a similar basic plan and have descended from a common form are said to be homologous, whether they have the same function or not. Since specialized walking legs, mouthparts, chelipeds, and swimmerets have all developed from a common biramous type but have become modified to perform different functions, they are all homologous to each other, a condition known as serial homology. Primitively limbs were all very similar, but during evolution of structural modifications, some branches have been reduced, some lost, some greatly altered, and some new parts added. Crayfishes and their allies possess the most elaborate serial homology in the animal kingdom, having 17 distinct but serially homologous types of appendages (Table 19-1).
Internal Features
The muscular and nervous systems and segmentation in the thorax and abdomen clearly show metamerism inherited from annelid-like ancestors, but there are marked modifications in other systems. Most changes involve concentration of parts in a particular region or else reduction or complete loss of parts, such as the intersepta.
Hemocoel: The major body space in arthropods is not the coelom but a blood-filled hemocoel. During embryonic development of most arthropods, vestigial coelomic cavities open within the mesoderm of at least some somites. These are soon obliterated or become continuous with the space between the developing mesodermal and ectodermal structures and the yolk. This space becomes the hemocoel and is thus not lined by a mesodermal peritoneum. In crustaceans the only coelomic compartments remaining are the end sacs of excretory organs and space around the gonads.
Muscular System: Striated muscles make up a considerable part of the body of most Crustacea. Muscles are usually arranged in antagonistic groups: flexors, which draw a part toward the body, and extensors, which extend it outward. The abdomen of a crayfish has powerful flexors (Figure 19-5), which are used when the animal swims backward—its best means of escape. Strong muscles on either side of the stomach control the mandibles.
Respiratory System: Respiratory gas exchange in smaller crustaceans occurs over thinner areas of cuticle (for example, in the legs) or the entire body, and specialized structures may be absent. Larger crustaceans have gills, which are delicate, featherlike projections with very thin cuticle. In decapods the sides of the carapace enclose the gill cavity, which is open anteriorly and ventrally (Figure 19-6). Gills may project from the pleural wall into the gill cavity, from the articulation of the thoracic legs with the body, or from the thoracic coxae. The latter two types are typical of crayfishes. The “bailer,” a part of the second maxilla, draws water over the gill filaments, into the gill cavity at the bases of the legs, and out of the gill cavity at the anterior.
Circulatory System: Crustaceans and other arthropods have an “open” or lacunar type of circulatory system. This means that there are no veins and no separation of blood from interstitial fluid, as there is in animals with closed systems. Hemolymph (blood) leaves the heart by way of arteries, circulates through the hemocoel, and returns to venous sinuses, or spaces, instead of veins before it reenters the heart. Annelids have a closed system, as do vertebrates.
A dorsal heart is the chief propulsive organ. It is a single-chambered sac of striated muscle. Hemolymph enters the heart from the surrounding pericardial sinus through paired ostia, with valves that prevent backflow into the sinus (Figure 19-6). From the heart hemolymph enters one or more arteries. Valves in the arteries prevent a backflow of hemolymph. Small arteries empty into tissue sinuses, which in turn often discharge into a large sternal sinus (Figure 19-6).
From there, afferent sinus channels carry hemolymph to the gills, if present, for oxygen and carbon dioxide exchange. Hemolymph then returns to the pericardial sinus by efferent channels (Figure 19-6).
Hemolymph in arthropods is largely colorless. It includes ameboid cells of at least two types. Hemocyanin, a copper-containing respiratory pigment, or hemoglobin, an iron-containing pigment, may be carried in solution. Hemolymph has the property of clotting, which prevents its loss in minor injuries. Some ameboid cells release a thrombinlike coagulant that precipitates clotting.
Excretory System: Excretory organs of adult crustaceans are a pair of tubular structures located in the ventral part of the head anterior to the esophagus (Figure 19-5). They are called antennal glands or maxillary glands, depending on whether they open at the base of the antennae or of the second maxillae. A few adult crustaceans have both. Excretory organs of decapods are antennal glands, also called green glands in this group. Crustaceans do not have malpighian tubules, the excretory organs of spiders and insects.
The end sac of the antennal gland, which is derived from an embryonic coelomic compartment, consists of a small vesicle (saccule) and a spongy mass called a labyrinth. The labyrinth connects by an excretory tubule to a dorsal bladder, which opens to the exterior by a pore on the ventral surface of the basal antennal segment (Figure 19-7). Hydrostatic pressure within the hemocoel provides force for filtration of fluid into the end sac. As filtrate passes through the excretory tubule and bladder, it is modified by resorption of salts, amino acids, glucose, and some water and is finally excreted as urine.
Excretion of nitrogenous wastes (mostly ammonia) takes place by diffusion across thin areas of cuticle, especially the gills, and the so-called excretory organs function principally to regulate ionic and osmotic composition of body fluids. Freshwater crustaceans, such as crayfishes, are constantly threatened with overdilution of their blood by water, which diffuses across the gills and other waterpermeable surfaces. The green glands, by forming a dilute, low-salt urine, act as an effective “flood-control” device. Some Na+ and Cl− are lost in the urine, but this loss is compensated by active absorption of dissolved salt by the gills. In marine crustaceans, such as lobsters and crabs, the kidney functions to adjust salt composition of hemolymph by selective modification of salt content of tubular urine. In these forms urine remains isosmotic to the blood.
Nervous and Sensory Systems: The nervous systems of crustaceans and annelids have much in common, although those of crustaceans have more fusion of ganglia (Figure 19-5). The brain is a pair of supraesophageal ganglia that supplies nerves to the eyes and two pairs of antennae. It is joined by connectives to the subesophageal ganglion, a fusion of at least five pairs of ganglia that supply nerves to the mouth, appendages, esophagus, and antennal glands. The double ventral nerve cord has a pair of ganglia for each somite and nerves serving the appendages, muscles, and other parts.
In addition to this central system, there may be a sympathetic nervous system associated with the digestive tract.
Crustaceans have better-developed sense organs than do annelids. The largest sense organs of crayfishes are eyes and statocysts. Widely distributed over the body are tactile hairs, delicate projections of cuticle that are especially abundant on chelae, mouthparts, and telson. The chemical senses of taste and smell are found in hairs on antennae, mouthparts, and other places.
A saclike statocyst, opening to the surface by a dorsal pore, is found on the basal segment of each first antenna of crayfishes. The statocyst contains a ridge that bears sensory hairs formed from the chitinous lining and grains of sand that serve as statoliths. Whenever the animal changes its position, corresponding changes in the position of the grains on the sensory hairs are relayed as stimuli to the brain, and the animal can adjust itself accordingly. Each molt (ecdysis) of cuticle results in loss of the cuticular lining of the statocyst and with it the sand grains. New grains are picked up through the dorsal pore after ecdysis.
The eyes in many crustaceans are compound, composed of many photoreceptor units called ommatidia (Figure 19-8). Covering the rounded surface of each eye is a transparent area of cuticle, the cornea, which is divided into many small squares or hexagons known as facets. These facets are the outer ends of the ommatidia. Each ommatidium behaves like a tiny eye and contains several kinds of cells arranged in a columnar fashion (Figure 19-8). Black pigment cells are found between adjacent ommatidia.
Movement of pigment in an arthropod compound eye permits it to adjust for different amounts of light. There are three sets of pigment cells in each ommatidium: distal retinal, proximal retinal, and reflecting; these are so arranged that they can form a more or less complete collar or sleeve around each ommatidium. For strong light or day adaptation the distal retinal pigment moves inward and meets the outward-moving proximal retinal pigment so that a complete pigment sleeve forms around the ommatidium (Figure 19-8). In this condition only rays that strike the cornea directly will reach the photoreceptor (retinular) cells, for each ommatidium is shielded from others. Thus each ommatidium will see only a limited area of the field of vision (a mosaic, or apposition, image). In dim light distal and proximal pigments separate so that the light rays, with the aid of reflecting pigment cells, have a chance to spread to adjacent ommatidia and to form a continuous, or superposition, image. This second type of vision is less precise but takes maximum advantage of the limited amount of light received.
Reproduction, Life Cycles, and Endocrine Function
Most crustaceans have separate sexes, and there are various specializations for copulation among different groups. Barnacles are monoecious but generally practice cross-fertilization. In some ostracods males are scarce, and reproduction is usually parthenogenetic. Most crustaceans brood their eggs in some manner: branchiopods and barnacles have special brood chambers, copepods have brood sacs attached to the sides of the abdomen (see Figure 19-19), and many malacostracans carry eggs and young attached to their abdominal appendages.
Crayfishes have direct development: there is no larval form. A tiny juvenile with the same form as the adult and a complete set of appendages and somites hatches from the egg. However, development is indirect in the majority of crustaceans, and a larva quite unlike the adult in structure and appearance hatches from the egg. Change from larva ultimately to an adult is metamorphosis. The primitive and most widely occurring larva in the Crustacea is the nauplius (Figure 19-9 and 19-23). Nauplii bear only three pairs of appendages: uniramous first antennules, biramous antennae, and biramous mandibles. All function as swimming appendages at this stage.
Subsequent development may involve a gradual change to the adult body form, and appendages and somites are added through a series of molts, or assumption of the adult form may involve more abrupt changes. For example, metamorphosis of a barnacle proceeds from a free-swimming nauplius to a larva with a bivalve carapace called a cyprid and finally to a sessile adult with calcareous plates.
Ecdysis: Ecdysis (ek´duh-sis) (Gr. ekdyein, to strip off), or molting, is necessary for the body to increase in size because the exoskeleton is nonliving and does not grow as the animal grows. Much of a crustacean’s functioning, including its reproduction, behavior, and many metabolic processes, is directly affected by the physiology of the molting cycle.
Cuticle, which is secreted by underlying epidermis, has several layers (Figure 19-10). The outermost is epicuticle, a very thin layer of lipidimpregnated protein. The bulk of cuticle is the several layers of procuticle: (1) exocuticle, which is just beneath the epicuticle and contains protein, calcium salts, and chitin; (2) endocuticle, which itself is composed of (3) a principal layer, which contains more chitin and less protein and is heavily calcified, and (4) an uncalcified membranous layer, a relatively thin layer of chitin and protein.
Some time before actual ecdysis, epidermal cells enlarge considerably. They separate from the membranous layer, secrete a new epicuticle, and begin secreting a new exocuticle (Figure 19-11). Enzymes are released into the area above the new epicuticle. These enzymes begin to dissolve old endocuticle, and the soluble products are resorbed and stored within the body of the crustacean. Some calcium salts are stored as gastroliths (mineral accretions) in the walls of the stomach. Finally, only exocuticle and epicuticle of the old cuticle remain, underlain by new epicuticle and new exocuticle. The animal swallows water, which it absorbs through its gut, and its blood volume increases greatly. Internal pressure causes the cuticle to split, and the animal pulls itself out of its old exoskeleton (Figure 19-12). Then follow a stretching of the still soft new cuticle, deposition of the new endocuticle, redeposition of the salvaged inorganic salts and other constituents, and hardening of the new cuticle. During the period of molting, the animal is defenseless and remains hidden away.
When a crustacean is young, ecdysis must occur frequently to allow growth, and the molting cycle is relatively short. As the animal approaches maturity, intermolt periods become progressively longer, and in some species molting ceases altogether. During intermolt periods, increase in tissue mass occurs as living tissue replaces water.
Hormonal Control of the Ecdysis Cycle
Although ecdysis is hormonally controlled, the cycle is often initiated by an environmental stimulus perceived by the central nervous system. Such stimuli may include temperature, day length, and humidity (in the case of land crabs). The signal from the central nervous system decreases production of a molt-inhibiting hormone by the X-organ. The X-organ is a group of neurosecretory cells in the medulla terminalis of the brain. In crayfishes and other decapods, the medulla terminalis is found in the eyestalk. The hormone is carried in the axons of the X-organ to the sinus gland (which itself is probably not glandular in function), also in the eyestalk, where it is released into the hemolymph.
A drop in the level of molt-inhibiing hormone promotes release of a molting hormone from the Y-organs. The Yorgans are beneath the epidermis near the adductor muscles of the mandibles, and they are homol-ogus to the prothoracic glands of insects, which produce the hormone ecdysone. The action of molting hormone is to initiate processes leading to ecdysis (proecdysis). Once initiated, the cycle proceeds automatically without further action of hormones from either the X- or Y-organs.
Other Endocrine Functions: Not only does removal of eyestalks accelerate molting, it was also found over 100 years ago that crustaceans whose eyestalks have been removed can no longer adjust body coloration to background conditions. Over 50 years ago it was discovered that the defect was caused not by loss of vision but by loss of hormones in the eyestalks. Body color of crustaceans is largely a result of pigments in special branched cells (chromatophores) in the epidermis.
Concentration of pigment granules in the center of the cells causes a lightening effect, and dispersal of pigment throughout the cells causes a darkening effect. Pigment behavior is controlled by hormones from neurosecretory cells in the eyestalk, as is migration of retinal pigment for light and dark adaptation in the eyes (Figure 19-8).
Release of neurosecretory material from the pericardial organs in the wall of the pericardium causes an increase in the rate and amplitude of the heartbeat.
Androgenic glands, first found in an amphipod (Orchestia, a common beach hopper), occur in male malacostracans. Unlike most other endocrine organs in crustaceans, these are not neurosecretory organs. Their secretion stimulates expression of male sexual characteristics. Young malacostracans have rudimentary androgenic glands, but in females these glands fail to develop. If they are artificially implanted in a female, her ovaries transform to testes and begin to produce sperm, and her appendages begin to take on male characteristics at the next molt. In isopods the androgenic glands are found in testes; in all other malacostracans they are between muscles of the coxopods of the last thoracic legs and partly attached near ends of the vasa deferentia. Although females do not possess organs similar to androgenic glands, their ovaries produce one or two hormones that influence secondary sexual characteristics.
Hormones that influence other body processes in Crustacea may be present, and evidence suggests that a neurosecretory substance produced in the eyestalk regulates the level of blood sugar.
Feeding Habits
Feeding habits and adaptations for feeding vary greatly among crustaceans. Many forms can shift from one type of feeding to another depending on environment and food availability, but all use the same fundamental set of mouthparts. Mandibles and maxillae function to ingest food; maxillipeds hold and crush food. In predators the walking legs, particularly chelipeds, serve in food capture.
Many crustaceans, both large and small, are predatory, and some have interesting adaptations for killing prey. One shrimplike form, Lygiosquilla, has on one of its walking legs a specialized digit that can be drawn into a groove and released suddenly to pierce passing prey. Pistol shrimps (Alpheus spp.) have an enormously enlarged chela that can be cocked like the hammer of a gun and snapped with a force that stuns their prey.
The food of suspension feeders ranges from plankton and detritus to bacteria. Predators consume larvae, worms, crustaceans, snails, and fishes. Scavengers eat dead animal and plant matter. Suspension feeders, such as the fairy shrimps, water fleas, and barnacles, use their legs, which bear a thick fringe of setae, to create water currents that sweep food particles through the setae. Mud shrimps (Upogebia spp.) use long setae on their first two pairs of thoracic appendages to strain food material from water circulated through their burrow by movements of their swimmerets.
Crayfishes have a two-part stomach (Figure 19-13). The first part contains a gastric mill in which food, already torn up by the mandibles, can be further ground up by three calcareous teeth into particles fine enough to pass through a setose filter in the second part; the food particles then pass into the intestine for chemical digestion.
General Nature of a Crustace
Crustaceans differ from other arthropods in a variety of ways, but the only truly distinguishing characteristic is that crustaceans are the only arthropods with two pairs of antennae. In addition to two pairs of antennae and a pair of mandibles, crustaceans have two pairs of maxillae on the head, followed by a pair of appendages on each body segment or somite. In some crustaceans not all somites bear appendages. All appendages, except perhaps the first antennae, are primitively biramous (two main branches), and at least some appendages of present-day adults show that condition. Organs specialized for respiration, if present, function as gills.
Most crustaceans have between 16 and 20 somites, but some forms have 60 somites or more. A larger number of somites is a primitive feature. The more derived condition is to have fewer segments and increased tagmatization. Major tagmata are head, thorax, and abdomen, but these are not homologous throughout the class (or even within some subclasses) because of varying degrees of fusion of somites, for example, as in the cephalothorax.
![]() |
Figure 19-1 Archetypical plan of Malacostraca. The two maxillae and three maxillipeds have been separated diagrammatically to illustrate the general plan. |
By far the largest group of crustaceans is class Malacostraca, which includes lobsters, crabs, shrimps, beach hoppers, sow bugs, and many others. These show a surprisingly constant arrangement of body segments and tagmata, which is considered the ancestral plan of the class (Figure 19-1). This typical body plan has a head of five (six embryonically) fused somites, a thorax of eight somites, and an abdomen of six somites (seven in a few species). At the anterior end is a nonsegmented rostrum and at the posterior end is a nonsegmented telson, which with the last abdominal somite and its uropods forms a tail fan in many forms.
In many crustaceans the dorsal cuticle of the head may extend posteriorly and around the sides of the animal to cover or be fused with some or all of the thoracic and abdominal somites. This covering is called a carapace. In some groups the carapace forms clamshell-like valves that cover most or all of the body. In decapods (including lobsters, shrimp, crabs, and others), the carapace covers the entire cephalothorax but not the abdomen.
Form and Function
Because of their size and easy availability, large crustaceans such as crayfishes have been studied more than other groups. They are also commonly studied in introductory laboratory courses. Therefore many of the comments that follow apply specifically to crayfishes and their relatives.
External Features
The bodies of crustaceans are covered with a secreted cuticle composed of chitin, protein, and calcareous material. The harder, heavy plates of larger crustaceans are particularly high in calcareous deposits. The hard protective covering is soft and thin at the joints between somites, allowing flexibility of movement. The carapace, if present, covers much or all of the cephalothorax; in decapods such as crayfishes, all head and thoracic segments are enclosed dorsally by the carapace. Each somite not enclosed by the carapace is covered by a dorsal cuticular plate, or tergum (Figure 19-2A), and a ventral transverse bar, the sternum, lies between the segmental appendages (Figure 19-2B). The abdomen terminates in a telson, which is not considered a somite and bears the anus. (The telson may be homologous to the annelid pygidium.)
![]() |
Figure 19-2 External structure of crayfishes. A, Dorsal view. B, Ventral view. |
![]() |
Figure 19-3 Parts of a biramous crustacean appendage (third maxilliped of a crayfish). |
Appendages: Members of classes Malacostraca (including crayfishes) and Remipedia typically have a pair of jointed appendages on each somite (Figure 19-3), although the abdominal somites in the other classes do not bear appendages. Considerable specialization is evident in appendages of derived crustaceans such as crayfishes. However, all are variations of the basic, biramous plan, illustrated by a crayfish appendage such as a maxilliped (a thoracic limb modified to become a head appendage) (Figures 19-3 and 19-4). The basal portion, or protopod, bears a lateral exopod and a medial endopod. The protopod is made up of one or two joints (basis and coxa), whereas the exopod and endopod have from one to several joints each. Some appendages, such as walking legs of crayfishes, have be-come secondarily uniramous. Medial or lateral processes sometimes occur on crustacean limbs, called endites and exites, respectively, and an exite on the protopod is called an epipod. Epipods are often modified as gills. Table 19-1 shows how the various appendages have become modified from the biramous plan to fit specific functions.
![]() |
Figure 19-4 Appendages of a crayfish showing how they have become modified from the basic biramous plan, as found in a swimmeret. Protopod, pink; endopod, purple; exopod, yellow. |
Structures that have a similar basic plan and have descended from a common form are said to be homologous, whether they have the same function or not. Since specialized walking legs, mouthparts, chelipeds, and swimmerets have all developed from a common biramous type but have become modified to perform different functions, they are all homologous to each other, a condition known as serial homology. Primitively limbs were all very similar, but during evolution of structural modifications, some branches have been reduced, some lost, some greatly altered, and some new parts added. Crayfishes and their allies possess the most elaborate serial homology in the animal kingdom, having 17 distinct but serially homologous types of appendages (Table 19-1).
![]() |
TABLE 19.1 |
Internal Features
The muscular and nervous systems and segmentation in the thorax and abdomen clearly show metamerism inherited from annelid-like ancestors, but there are marked modifications in other systems. Most changes involve concentration of parts in a particular region or else reduction or complete loss of parts, such as the intersepta.
Hemocoel: The major body space in arthropods is not the coelom but a blood-filled hemocoel. During embryonic development of most arthropods, vestigial coelomic cavities open within the mesoderm of at least some somites. These are soon obliterated or become continuous with the space between the developing mesodermal and ectodermal structures and the yolk. This space becomes the hemocoel and is thus not lined by a mesodermal peritoneum. In crustaceans the only coelomic compartments remaining are the end sacs of excretory organs and space around the gonads.
Muscular System: Striated muscles make up a considerable part of the body of most Crustacea. Muscles are usually arranged in antagonistic groups: flexors, which draw a part toward the body, and extensors, which extend it outward. The abdomen of a crayfish has powerful flexors (Figure 19-5), which are used when the animal swims backward—its best means of escape. Strong muscles on either side of the stomach control the mandibles.
![]() |
Figure 19-5 Internal structure of a male crayfish. |
Respiratory System: Respiratory gas exchange in smaller crustaceans occurs over thinner areas of cuticle (for example, in the legs) or the entire body, and specialized structures may be absent. Larger crustaceans have gills, which are delicate, featherlike projections with very thin cuticle. In decapods the sides of the carapace enclose the gill cavity, which is open anteriorly and ventrally (Figure 19-6). Gills may project from the pleural wall into the gill cavity, from the articulation of the thoracic legs with the body, or from the thoracic coxae. The latter two types are typical of crayfishes. The “bailer,” a part of the second maxilla, draws water over the gill filaments, into the gill cavity at the bases of the legs, and out of the gill cavity at the anterior.
Circulatory System: Crustaceans and other arthropods have an “open” or lacunar type of circulatory system. This means that there are no veins and no separation of blood from interstitial fluid, as there is in animals with closed systems. Hemolymph (blood) leaves the heart by way of arteries, circulates through the hemocoel, and returns to venous sinuses, or spaces, instead of veins before it reenters the heart. Annelids have a closed system, as do vertebrates.
A dorsal heart is the chief propulsive organ. It is a single-chambered sac of striated muscle. Hemolymph enters the heart from the surrounding pericardial sinus through paired ostia, with valves that prevent backflow into the sinus (Figure 19-6). From the heart hemolymph enters one or more arteries. Valves in the arteries prevent a backflow of hemolymph. Small arteries empty into tissue sinuses, which in turn often discharge into a large sternal sinus (Figure 19-6).
From there, afferent sinus channels carry hemolymph to the gills, if present, for oxygen and carbon dioxide exchange. Hemolymph then returns to the pericardial sinus by efferent channels (Figure 19-6).
![]() |
Figure 19-6 Diagrammatical cross section through heart region of a crayfish showing direction of blood flow in this “open” blood system. Heart pumps blood to body tissues through arteries, which empty into tissue sinuses. Returning blood enters sternal sinus, then goes through gills for gas exchange, and finally back to pericardial sinus by efferent channels. Note absence of veins. |
Hemolymph in arthropods is largely colorless. It includes ameboid cells of at least two types. Hemocyanin, a copper-containing respiratory pigment, or hemoglobin, an iron-containing pigment, may be carried in solution. Hemolymph has the property of clotting, which prevents its loss in minor injuries. Some ameboid cells release a thrombinlike coagulant that precipitates clotting.
![]() |
Figure 19-7 Scheme of antennal gland (green gland) of crayfishes. (In natural position organ is much folded.) Some crustaceans lack a labyrinth, and the excretory tubule (nephridial canal) is a muchcoiled tube. |
Excretory System: Excretory organs of adult crustaceans are a pair of tubular structures located in the ventral part of the head anterior to the esophagus (Figure 19-5). They are called antennal glands or maxillary glands, depending on whether they open at the base of the antennae or of the second maxillae. A few adult crustaceans have both. Excretory organs of decapods are antennal glands, also called green glands in this group. Crustaceans do not have malpighian tubules, the excretory organs of spiders and insects.
The end sac of the antennal gland, which is derived from an embryonic coelomic compartment, consists of a small vesicle (saccule) and a spongy mass called a labyrinth. The labyrinth connects by an excretory tubule to a dorsal bladder, which opens to the exterior by a pore on the ventral surface of the basal antennal segment (Figure 19-7). Hydrostatic pressure within the hemocoel provides force for filtration of fluid into the end sac. As filtrate passes through the excretory tubule and bladder, it is modified by resorption of salts, amino acids, glucose, and some water and is finally excreted as urine.
Excretion of nitrogenous wastes (mostly ammonia) takes place by diffusion across thin areas of cuticle, especially the gills, and the so-called excretory organs function principally to regulate ionic and osmotic composition of body fluids. Freshwater crustaceans, such as crayfishes, are constantly threatened with overdilution of their blood by water, which diffuses across the gills and other waterpermeable surfaces. The green glands, by forming a dilute, low-salt urine, act as an effective “flood-control” device. Some Na+ and Cl− are lost in the urine, but this loss is compensated by active absorption of dissolved salt by the gills. In marine crustaceans, such as lobsters and crabs, the kidney functions to adjust salt composition of hemolymph by selective modification of salt content of tubular urine. In these forms urine remains isosmotic to the blood.
Nervous and Sensory Systems: The nervous systems of crustaceans and annelids have much in common, although those of crustaceans have more fusion of ganglia (Figure 19-5). The brain is a pair of supraesophageal ganglia that supplies nerves to the eyes and two pairs of antennae. It is joined by connectives to the subesophageal ganglion, a fusion of at least five pairs of ganglia that supply nerves to the mouth, appendages, esophagus, and antennal glands. The double ventral nerve cord has a pair of ganglia for each somite and nerves serving the appendages, muscles, and other parts.
In addition to this central system, there may be a sympathetic nervous system associated with the digestive tract.
Crustaceans have better-developed sense organs than do annelids. The largest sense organs of crayfishes are eyes and statocysts. Widely distributed over the body are tactile hairs, delicate projections of cuticle that are especially abundant on chelae, mouthparts, and telson. The chemical senses of taste and smell are found in hairs on antennae, mouthparts, and other places.
A saclike statocyst, opening to the surface by a dorsal pore, is found on the basal segment of each first antenna of crayfishes. The statocyst contains a ridge that bears sensory hairs formed from the chitinous lining and grains of sand that serve as statoliths. Whenever the animal changes its position, corresponding changes in the position of the grains on the sensory hairs are relayed as stimuli to the brain, and the animal can adjust itself accordingly. Each molt (ecdysis) of cuticle results in loss of the cuticular lining of the statocyst and with it the sand grains. New grains are picked up through the dorsal pore after ecdysis.
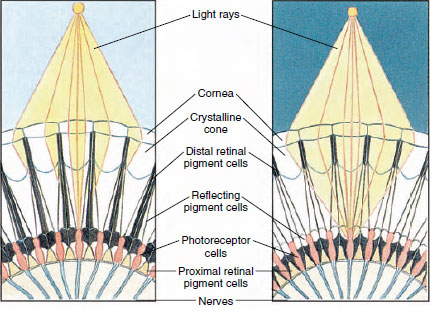
Figure 19-8 Portion of compound eye of an arthropod showing migration of pigment in ommatidia for day and night vision. Five ommatidia are represented in each diagram. In daytime each ommatidium is surrounded by a dark pigment collar so that each ommatidium is stimulated only by light rays that enter its own cornea (mosaic vision); in nighttime, pigment forms incomplete collars and light rays can spread to adjacent ommatidia (continuous, or superposition, image). |
The eyes in many crustaceans are compound, composed of many photoreceptor units called ommatidia (Figure 19-8). Covering the rounded surface of each eye is a transparent area of cuticle, the cornea, which is divided into many small squares or hexagons known as facets. These facets are the outer ends of the ommatidia. Each ommatidium behaves like a tiny eye and contains several kinds of cells arranged in a columnar fashion (Figure 19-8). Black pigment cells are found between adjacent ommatidia.
Movement of pigment in an arthropod compound eye permits it to adjust for different amounts of light. There are three sets of pigment cells in each ommatidium: distal retinal, proximal retinal, and reflecting; these are so arranged that they can form a more or less complete collar or sleeve around each ommatidium. For strong light or day adaptation the distal retinal pigment moves inward and meets the outward-moving proximal retinal pigment so that a complete pigment sleeve forms around the ommatidium (Figure 19-8). In this condition only rays that strike the cornea directly will reach the photoreceptor (retinular) cells, for each ommatidium is shielded from others. Thus each ommatidium will see only a limited area of the field of vision (a mosaic, or apposition, image). In dim light distal and proximal pigments separate so that the light rays, with the aid of reflecting pigment cells, have a chance to spread to adjacent ommatidia and to form a continuous, or superposition, image. This second type of vision is less precise but takes maximum advantage of the limited amount of light received.
Reproduction, Life Cycles, and Endocrine Function
Most crustaceans have separate sexes, and there are various specializations for copulation among different groups. Barnacles are monoecious but generally practice cross-fertilization. In some ostracods males are scarce, and reproduction is usually parthenogenetic. Most crustaceans brood their eggs in some manner: branchiopods and barnacles have special brood chambers, copepods have brood sacs attached to the sides of the abdomen (see Figure 19-19), and many malacostracans carry eggs and young attached to their abdominal appendages.
![]() |
Figure 19-9 Life cycle of a Gulf shrimp Penaeus. Penaeids spawn at depths of 40 to 90 m. The young larval forms are planktonic and move inshore to water of lower salinity to develop as juveniles. Older shrimp return to deeper water offshore. |
Crayfishes have direct development: there is no larval form. A tiny juvenile with the same form as the adult and a complete set of appendages and somites hatches from the egg. However, development is indirect in the majority of crustaceans, and a larva quite unlike the adult in structure and appearance hatches from the egg. Change from larva ultimately to an adult is metamorphosis. The primitive and most widely occurring larva in the Crustacea is the nauplius (Figure 19-9 and 19-23). Nauplii bear only three pairs of appendages: uniramous first antennules, biramous antennae, and biramous mandibles. All function as swimming appendages at this stage.
![]() |
Figure 19-10 Structure of crustacean cuticle. |
Subsequent development may involve a gradual change to the adult body form, and appendages and somites are added through a series of molts, or assumption of the adult form may involve more abrupt changes. For example, metamorphosis of a barnacle proceeds from a free-swimming nauplius to a larva with a bivalve carapace called a cyprid and finally to a sessile adult with calcareous plates.
Ecdysis: Ecdysis (ek´duh-sis) (Gr. ekdyein, to strip off), or molting, is necessary for the body to increase in size because the exoskeleton is nonliving and does not grow as the animal grows. Much of a crustacean’s functioning, including its reproduction, behavior, and many metabolic processes, is directly affected by the physiology of the molting cycle.
Cuticle, which is secreted by underlying epidermis, has several layers (Figure 19-10). The outermost is epicuticle, a very thin layer of lipidimpregnated protein. The bulk of cuticle is the several layers of procuticle: (1) exocuticle, which is just beneath the epicuticle and contains protein, calcium salts, and chitin; (2) endocuticle, which itself is composed of (3) a principal layer, which contains more chitin and less protein and is heavily calcified, and (4) an uncalcified membranous layer, a relatively thin layer of chitin and protein.
Some time before actual ecdysis, epidermal cells enlarge considerably. They separate from the membranous layer, secrete a new epicuticle, and begin secreting a new exocuticle (Figure 19-11). Enzymes are released into the area above the new epicuticle. These enzymes begin to dissolve old endocuticle, and the soluble products are resorbed and stored within the body of the crustacean. Some calcium salts are stored as gastroliths (mineral accretions) in the walls of the stomach. Finally, only exocuticle and epicuticle of the old cuticle remain, underlain by new epicuticle and new exocuticle. The animal swallows water, which it absorbs through its gut, and its blood volume increases greatly. Internal pressure causes the cuticle to split, and the animal pulls itself out of its old exoskeleton (Figure 19-12). Then follow a stretching of the still soft new cuticle, deposition of the new endocuticle, redeposition of the salvaged inorganic salts and other constituents, and hardening of the new cuticle. During the period of molting, the animal is defenseless and remains hidden away.
![]() |
|
![]() |
![]() |
Figure 19-12 Molting sequence in a lobster, Homarus americanus. A, Membrane between carapace and abdomen ruptures, and carapace begins slow elevation. This step may take up to 2 hours. B and C, Head, thorax, and finally abdomen withdraw. This process usually takes no more than 15 minutes. Immediately after ecdysis, chelipeds are desiccated and body is very soft. Lobster continues rapid absorption of water so that within 12 hours the body increases about 20% in length and 50% in weight. Tissue water will be replaced by protein in succeeding weeks. |
Figure 19-11 Cuticle secretion and resorption in ecdysis. |
When a crustacean is young, ecdysis must occur frequently to allow growth, and the molting cycle is relatively short. As the animal approaches maturity, intermolt periods become progressively longer, and in some species molting ceases altogether. During intermolt periods, increase in tissue mass occurs as living tissue replaces water.
Hormonal Control of the Ecdysis Cycle
Although ecdysis is hormonally controlled, the cycle is often initiated by an environmental stimulus perceived by the central nervous system. Such stimuli may include temperature, day length, and humidity (in the case of land crabs). The signal from the central nervous system decreases production of a molt-inhibiting hormone by the X-organ. The X-organ is a group of neurosecretory cells in the medulla terminalis of the brain. In crayfishes and other decapods, the medulla terminalis is found in the eyestalk. The hormone is carried in the axons of the X-organ to the sinus gland (which itself is probably not glandular in function), also in the eyestalk, where it is released into the hemolymph.
A drop in the level of molt-inhibiing hormone promotes release of a molting hormone from the Y-organs. The Yorgans are beneath the epidermis near the adductor muscles of the mandibles, and they are homol-ogus to the prothoracic glands of insects, which produce the hormone ecdysone. The action of molting hormone is to initiate processes leading to ecdysis (proecdysis). Once initiated, the cycle proceeds automatically without further action of hormones from either the X- or Y-organs.
Other Endocrine Functions: Not only does removal of eyestalks accelerate molting, it was also found over 100 years ago that crustaceans whose eyestalks have been removed can no longer adjust body coloration to background conditions. Over 50 years ago it was discovered that the defect was caused not by loss of vision but by loss of hormones in the eyestalks. Body color of crustaceans is largely a result of pigments in special branched cells (chromatophores) in the epidermis.
Concentration of pigment granules in the center of the cells causes a lightening effect, and dispersal of pigment throughout the cells causes a darkening effect. Pigment behavior is controlled by hormones from neurosecretory cells in the eyestalk, as is migration of retinal pigment for light and dark adaptation in the eyes (Figure 19-8).
Release of neurosecretory material from the pericardial organs in the wall of the pericardium causes an increase in the rate and amplitude of the heartbeat.
Androgenic glands, first found in an amphipod (Orchestia, a common beach hopper), occur in male malacostracans. Unlike most other endocrine organs in crustaceans, these are not neurosecretory organs. Their secretion stimulates expression of male sexual characteristics. Young malacostracans have rudimentary androgenic glands, but in females these glands fail to develop. If they are artificially implanted in a female, her ovaries transform to testes and begin to produce sperm, and her appendages begin to take on male characteristics at the next molt. In isopods the androgenic glands are found in testes; in all other malacostracans they are between muscles of the coxopods of the last thoracic legs and partly attached near ends of the vasa deferentia. Although females do not possess organs similar to androgenic glands, their ovaries produce one or two hormones that influence secondary sexual characteristics.
Hormones that influence other body processes in Crustacea may be present, and evidence suggests that a neurosecretory substance produced in the eyestalk regulates the level of blood sugar.
Feeding Habits
Feeding habits and adaptations for feeding vary greatly among crustaceans. Many forms can shift from one type of feeding to another depending on environment and food availability, but all use the same fundamental set of mouthparts. Mandibles and maxillae function to ingest food; maxillipeds hold and crush food. In predators the walking legs, particularly chelipeds, serve in food capture.
Many crustaceans, both large and small, are predatory, and some have interesting adaptations for killing prey. One shrimplike form, Lygiosquilla, has on one of its walking legs a specialized digit that can be drawn into a groove and released suddenly to pierce passing prey. Pistol shrimps (Alpheus spp.) have an enormously enlarged chela that can be cocked like the hammer of a gun and snapped with a force that stuns their prey.
The food of suspension feeders ranges from plankton and detritus to bacteria. Predators consume larvae, worms, crustaceans, snails, and fishes. Scavengers eat dead animal and plant matter. Suspension feeders, such as the fairy shrimps, water fleas, and barnacles, use their legs, which bear a thick fringe of setae, to create water currents that sweep food particles through the setae. Mud shrimps (Upogebia spp.) use long setae on their first two pairs of thoracic appendages to strain food material from water circulated through their burrow by movements of their swimmerets.
Crayfishes have a two-part stomach (Figure 19-13). The first part contains a gastric mill in which food, already torn up by the mandibles, can be further ground up by three calcareous teeth into particles fine enough to pass through a setose filter in the second part; the food particles then pass into the intestine for chemical digestion.
![]() |
Figure 19-13 Malacostracan stomach showing gastric “mill” and directions of food movements. Mill has chitinous ridges, or teeth, for mastication, and setae for straining food before it passes into the pyloric stomach. |