Structure of ribosomes
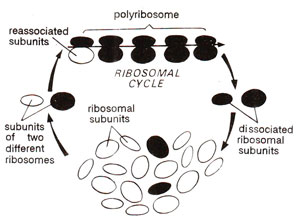
Fig. 31.8. Ribosomal cycle showing formation of polyribosome.
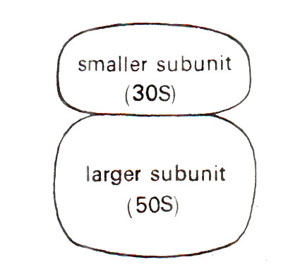
Fig. 31.9. A prokaryotic ribosome showing two subunits.

Fig. 31.10. Association of eukaryotic ribosomes or their subunits as dependent on Mg++ ion concentration.
Ribosomes are also-often observed in clusters and under these conditions are known as 'polyribosomes', or 'polysomes' rarely also known as ergosomes. The polyribosomes consist of those ribosomes which are actively engaged in protein synthesis and are held together by a string known as mRNA (Fig. 31.8). The dissociation of ribosomes in their subunits and their association to form ribosomes again, are related to their function in protein synthesis. Molecular organization of ribosomes and their function has been studied more intensively in prokaryotes than in eukaryotes, so that their structure and function will be discussed in this section in prokaryotes and important differences found in eukaryotes will be outlined.
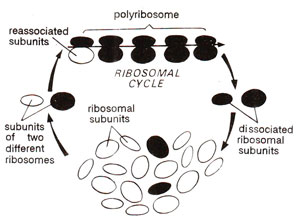
Fig. 31.8. Ribosomal cycle showing formation of polyribosome.
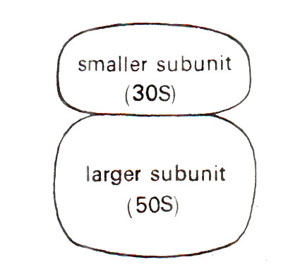
Fig. 31.9. A prokaryotic ribosome showing two subunits.

Fig. 31.10. Association of eukaryotic ribosomes or their subunits as dependent on Mg++ ion concentration.
In bacterial cells as well as in chloroplasts of higher plants, sedimentation coefficient of complete ribosomes is 70S, that of smaller subunit is 30S and that of bigger subunit is 50S. In higher organisms, sedimentation coefficient of ribosomes is 80S and those of subunits are 40S and 60S (Table 31.3). The ribosomes in mitochondria of higher organisms vary in size. In plant mitochondria, they are only little smaller than the ribosomes in surrounding cytoplasm (in maize mitochondria, 78S). In mitochondria of lower eukaryotes (e.g. fungi), these are slightly larger than in E. coli (73S in yeast), and in mammalian mitochondria they are much smaller (60S in Xenopus and human mitochondria).

Fig. 31.11. Diagrammatic representation of three-dimensional model of E. coli ribosome shown in two different orientations (A and B). (Redrawn from Sci. Amer., Vol. 245, 1981).

Fig. 31.12. Photographs of a model of a ribosome-side and front views (showing 30S subunit in black and 50S subunit in white; Photographs from M. Boublik, Roche Institute of Molecular Biology).
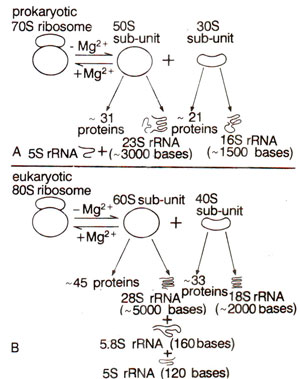
Fig. 31.13. Different RNA and protein components of prokaryote and eukaryote ribosomes.

Fig. 31.14. Secondary structure of a part (3' end) of 16S rRNA showing double stranded regions.

Fig. 31.15. A diagrammatic representation of different steps involved in reassociation of E. coli ribosome from its rRNA and protein units (S1-S21 = proteins of smaller subunit : L1-L33 = proteins of larger subunit). (redrawn from Watson—Molecular Biology of the Gene-1975).

Fig. 31.16. Map of proteins in the ribosome showing their position in small subunit (left) and the large subunit (right).

Fig. 31.17. Different active centres in the complete 70S ribosome and its two subunits (30S and 50S) in E. coli.

Fig. 31.18. Electron micrograph showing synthesis of rRNA on nucleolar genes isolated from an oocyte of newt, Triturus viredescens. (O.L. Miller and B.R. Beatty "Portrait of a gene").
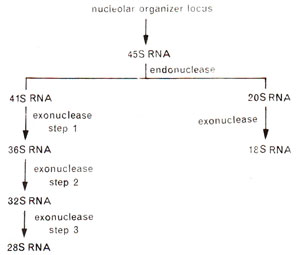
Fig. 31.19. A schematic representation showing synthesis of 28S and 18S rRNA from nucleolar organizer loci.
(b) Chemical composition. The major components of ribosomes are RNA and proteins. In an entire ribosome, rRNA makes 66% part in prokaryotes and 60% in eukaryotes. In subunits, the proportion of rRNA is larger in larger subunit (70% in prokaryotes and 65% in eukaryotes) than in the smaller subunit (60% in prokaryotes and 50% in eukaryotes). There is no lipid content in ribosomes. The positive charges are not enough to neutralize the negative charges of RNA, so that the ribosomes are strongly negative, binding cations and basic dyes.
The 30S and 50S subunits in prokaryotes may be dissociated to give rise to inactive core particles having sedimentation coefficients of 23S and 40S respectively. These particles (23S and 40S) contain RNA (16S and 23S respectively) and some core proteins. These RNAs in eukaryotes form 28S and 18S particles instead of 23S and 16S as observed in prokaryotes (Table 31.3; Fig. 31.13). Besides these core proteins, there are other proteins called split proteins (SP) in both subunits, which can be further fractionated into acidic and basic proteins. In all, 21 proteins were isolated in smaller subunit (30S) of E. coli ribosome. These are designated as SI to S21. Similarly, in larger subunit (50S) of E. coli ribosome, 31 different proteins are designated as LI to L34 (due to early misassignment, these are not designated LI to L31). In eukaryotes, however, there are 33 proteins in the smaller subunit (40S) and 45 proteins in larger subunit (60S). The sequences of bases in rRNAs and those of amino acids in most ribosomal proteins have also been determined. The organization of these proteins in ribosomes and their relative roles were studied in great detail during 1980s.
When RNA of ribosome was analysed in detail, it could be demonstrated that 60 per cent of RNA is helical (double stranded) and contains paired bases due to intrastrand complementary base pairing (Fig. 31.14). In Escherichia coli, the sequences of 23S and 16S RNAs have been analysed and were found to be specific showing no homology. The larger subunit contains an additional RNA (5S both in prokaryotes and eukaryotes and 5.8S in eukaryotes only). Complete sequence of 5S RNA is now known in E. coli and many eukaryotes and it was shown that 5S RNA is needed for correct assembly of larger subunit.
Dissociation and re constitution. In recent years, it has been possible to dissociate the ribosomal proteins completely, which could be followed by reconstitution of their structure and function. While dissociation is achieved by gradient centrifugation, reconstitution is brought about by adding.rRNAs to corresponding proteins. The steps involved in reconstitution are shown in Figure 31.15.
Reconstitution experiment showed that while some ribosomal proteins play structural role, others play specific functional roles. Location of important proteins and rRNA of ribosomes are shown in Figure 31.16 and several active centres are shown in Figure 31.17. For rRNA, it has been shown that there are common sequences in mRNAs, which pair with a sequence of rRNA, thus helping in mRNA-ribosome binding. It has also been shown that ribosomes are able to distinguish between the entry of correct and incorrect amino acyl-tRNA-EF-Tu-GTP complexes. The three proteins, which help in this function are S-4, S-5 and S-12, as shown with the help of mutations, which make the ribosomes resistant to streptomycin. It has been shown that in susceptible ribosomes, misreading occurs in the presence of streptomycin, but this misreading is reduced in the resistant ribosomes, which have alterations in either protein S-4 or S-5 or S-12.
Biogenesis. The complex organization of ribosome as described earlier, involves specific rRNA (ribosomal RNA) and 50 or more proteins, suggesting that atleast more than 50 genes may be involved in their formation.

Fig. 31.11. Diagrammatic representation of three-dimensional model of E. coli ribosome shown in two different orientations (A and B). (Redrawn from Sci. Amer., Vol. 245, 1981).

Fig. 31.12. Photographs of a model of a ribosome-side and front views (showing 30S subunit in black and 50S subunit in white; Photographs from M. Boublik, Roche Institute of Molecular Biology).
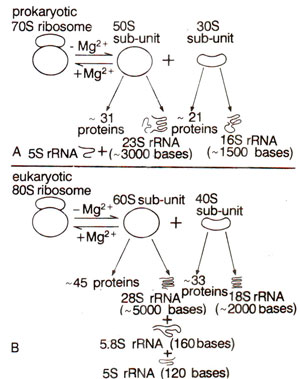
Fig. 31.13. Different RNA and protein components of prokaryote and eukaryote ribosomes.

Fig. 31.14. Secondary structure of a part (3' end) of 16S rRNA showing double stranded regions.

Fig. 31.15. A diagrammatic representation of different steps involved in reassociation of E. coli ribosome from its rRNA and protein units (S1-S21 = proteins of smaller subunit : L1-L33 = proteins of larger subunit). (redrawn from Watson—Molecular Biology of the Gene-1975).

Fig. 31.16. Map of proteins in the ribosome showing their position in small subunit (left) and the large subunit (right).

Fig. 31.17. Different active centres in the complete 70S ribosome and its two subunits (30S and 50S) in E. coli.

Fig. 31.18. Electron micrograph showing synthesis of rRNA on nucleolar genes isolated from an oocyte of newt, Triturus viredescens. (O.L. Miller and B.R. Beatty "Portrait of a gene").
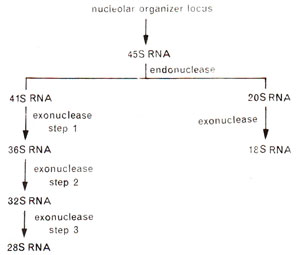
Fig. 31.19. A schematic representation showing synthesis of 28S and 18S rRNA from nucleolar organizer loci.