Introduction
Oils and fats tend to be the predominant energy reserves in mobile organisms
because of their high energy value per unit weight. Plants, given a sessile lifestyle,
limit oil production primarily to portable reproductive structures. Nevertheless,
more than 120 million metric tons of vegetable oil reach world markets per year
(United States Department of Agriculture, Foreign Agricultural Service, 2007).
Oilseeds such as soybean, sunflower, and rapeseed are the major oil crops in
temperate regions, although fruits of olive and especially of oil palm are significant
sources on a world basis (Table 7.1).
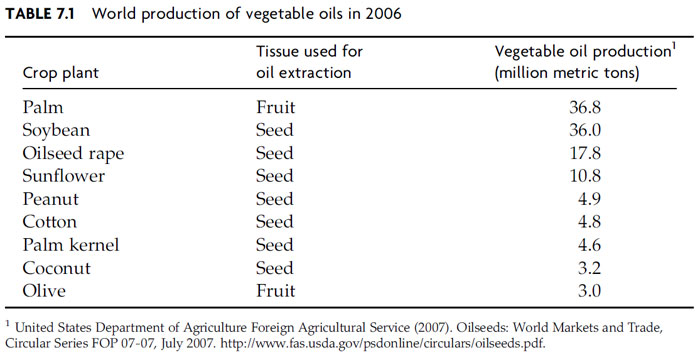 |
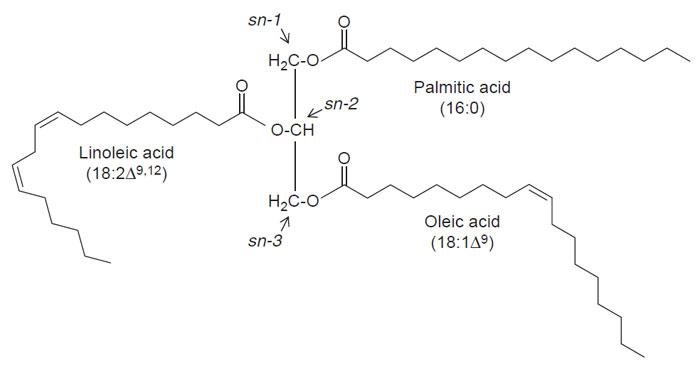 |
FIGURE 7.1 Structure of a typical triacylglycerol (TAG) molecule of vegetable oil. A TAG
molecule consists of fatty acids attached by ester linkages to each of the three stereospecific or sn
positions of a glycerol backbone. As shown, the sn-2 position of a typical plant TAG is occupied by
an unsaturated fatty acid. Saturated fatty acids generally occupy only the sn-1 or sn-3 positions,
but unsaturated fatty acids can be found at any of the three stereospecific positions. |
 |
FIGURE 7.2 Structure of linoleic acid. This
structure illustrates the basis for the shorthand
notation that is often used for fatty acids.
The 18:2Δ9,12 abbreviation indicates that linoleic
acid
contains 18 carbon atoms and 2 double
bonds, which are located at the C-9 and C-12
atoms relative
to the carboxyl end of the fatty
acid. Linoleic acid is often referred to as an ω-6
fatty acid,
which indicates that the last double
bond is positioned six carbon atoms from the
methyl end
of the fatty acid. Vegetable oils rich in
linoleic acid, such as soybean oil, are sometimes
called
ω-6 oils. |
At the molecular level, the typical oil molecule is a triacylglycerol (TAG), a
glycerol molecule with a fatty acid esterified to each of the three hydroxyl groups
(Fig. 7.1). The three carbon atoms of the glycerol backbone of TAG are referred to
using the stereospecific numbering system as
sn-1,
sn-2, and
sn-3 (Fig. 7.1). As
indicated by this nomenclature, the three carbons of glycerol are stereochemically
distinct. It is the fatty acid composition that determines the physical characteristics
of a given oil. For example, a sufficient proportion of saturated fatty acids, which
lack carbon–carbon double bonds, can raise the melting point of an oil until it is
solid at room temperature, as required in some baked goods. Palmitic acid,
abbreviated 16:0 because it has 16 carbons and 0 double bonds, is the most
abundant of the saturated fatty acids in plants, although at least some stearic
acid (18:0) occurs in most edible oils (Table 7.2). The unsaturated fatty acids of typical plant oils feature one or more
cis-double bonds, which introduce kinks into
the fatty acid chain and increase fluidity more effectively than would
trans-double
bonds. Oleic acid (18:1Δ
9), the most prominent monounsaturated fatty acid,
has a
cis-double bond nine carbons from its carboxyl terminus (see Fig. 7.2 for
explanation of numerical fatty acid nomenclature). It can comprise 65–85% of the
olive (
Olea) oil for which it was named, but contributes a mere 20% of traditional
sunflower or soybean oils (Gunstone
et al., 2007). Thus, high oleic acid seed oils
mimicking the qualities of olive oil as a cooking and salad oil are under development.
Plant oils are also important sources of polyunsaturated fatty acids including
linoleic acid (18:2Δ
9,12; Fig. 7.2) and α-linolenic acid (18:3Δ
9,12,15). Since increasing unsaturation decreases oxidative stability, oils high in 18:3 become
rancid quickly and are unsuitable for frying. However, both linoleic and
α-linolenic acids are essential to the human diet. Finally, some qualities of vegetable
oils reflect the arrangement of fatty acids on glycerol as well as absolute fatty
acid composition. For example, the positive ‘‘mouthfeel’’ of cocoa butter is largely
attributed to TAG having saturated fatty acids at positions 1 and 3, but 18:1Δ
9 at
position 2 (Jandacek, 1992). The positional distribution of fatty acids in dietary
TAG also has clinical implications (Kubow, 1996).
Although vegetable oils are primarily used in foods, they also serve as
industrial feedstocks (Table 7.3). A few oils are targeted entirely to such uses.
Highly unsaturated ‘‘drying oils’’ such as linseed oil are desirable for paints and
coatings; lauric acid (12:0) in coconut and palm kernel oil is a vital component of
soaps and detergents; castor oil, which contains the unusual hydroxy-fatty acid
ricinoleic acid (12-hydroxy-18:1Δ
9), is used for certain plastics and lubricants; and
high erucate (22:1Δ
13) rapeseed oil contains the raw material for Nylon 1313
and slip agents used in the manufacture of sheet plastic. Edible oils may likewise
serve industrial purposes. For example, in the United States, 12% of soybean oil
is currently channeled to products ranging from lubricants and biodiesel fuels
to inks, polyurethane, and candles (American Soybean Association, 2007). As
petroleum stocks dwindle, it is likely that vegetable oils will play a greater
industrial role.
 |
In addition to control of oil composition, improvement of total yield of oil
crops is a major goal of breeders and molecular biologists. To some extent, such
improvement can involve parameters beyond the scope of this discussion. Flower
number and seed set, disease resistance and fruit or seed size are only a few
examples of factors indirectly affecting oil production. At a more direct level,
scientists are attempting to identify control points for carbon flux into fatty
acids, factors influencing partitioning of fatty acids between structural lipids
and TAG, and regulatory elements determining overall expression of lipid
biosynthesis genes.