Molecular Biology of Plant Pathways / Metabolic Engineering of the Content and Fatty Acid Composition of Vegetable Oils
Metabolic Engineering of High and Low Polyunsaturated Vegetable Oils
Alteration of the a-linolenic content of seed oils is an important biotechnological
target. This fatty acid is a very minor component of the seed oil of a number of
crops, including corn, sunflower, peanut, and canola. α-Linolenic acid, however,
accounts for nearly 10% of soybean oil and over 50% of linseed (or flax) oil. The
three double bonds of this fatty acid make it particularly prone to oxidation. This
is an undesirable property for food processing as the oxidation products of
α-linolenic result in rancidity and reduced shelf life. Conversely, the oxidative
instability of α-linolenic acid is an essential property for the use of vegetable oils
such as linseed oil in drying oil applications. The free radicals generated from
oxidation of α-linolenic acid-rich oils result in the enhanced polymerization
(or ‘‘drying’’) of paint, ink, and other coating materials.
The α-linolenic acid content of seed oils can be increased or decreased by altering
the expression of genes for FAD3, the ER Δ
15-linoleic acid desaturase (Table 7.4 and
Fig. 7.4). As described above, FAD3 catalyzes the conversion of linoleic acid to
a-linolenic acid. Transgenic expression of the
A. thaliana FAD3 gene to high levels
using a strong seed-specific promoter has been shown to increase the α-linolenic
acid content to >50% of
A. thaliana seed oil, which is comparable to the proportion
found in linseed oil (Yadav
et al., 1993).
Downregulation of FAD3 expression in
seeds can be achieved through transgenic approaches or by the generation of
mutants. The development of FAD3 mutants with good agronomic performance
has been particularly effective in soybean. Mutants with as little as 1–3% α-linolenic
acid in their seed oil have been reported (Ross
et al., 2000). These mutants do not
display any significant reductions in seed yield (Ross
et al., 2000). It is also notable
that transgenic suppression of
FAD2 genes in soybean likewise yields oils with 2–3%
of a-linolenic acid. This phenotype is due to the large decrease in the linoleate
substrate pool for the Δ
15-linoleic acid desaturase (Buhr
et al., 2002; Kinney, 1996).
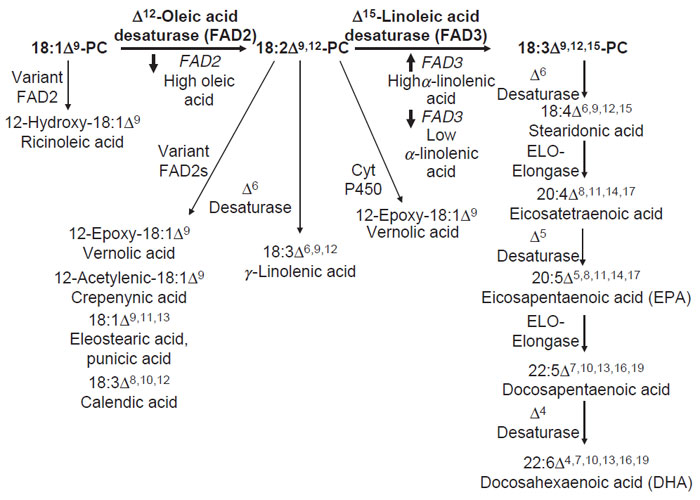 |
FIGURE 7.4 Examples of commercially important fatty acid modification reactions that can
occur in the ER of seeds. The Δ12-oleic acid desaturase or FAD2 and the Δ15-linoleic acid desaturase
or FAD3 commonly occur in seeds. By up- or downregulating the expression of FAD2 and FAD3 genes, the relative levels of vegetable oil unsaturation can be altered. Variant forms of enzymes
such as FAD2, cytochrome P450 monoxygenase, and cytochrome b5-fusion desaturases can be
transgenically expressed in existing oilseeds to produce unusual fatty acids such as ricinoleic,
vernolic, and GLAs. In addition, desaturases and ELO elongases from sources including mosses,
fungi, and algae can be engineered into oilseed crops to produce the nutritionally important longchain
polyunsaturated fatty acids eicosapentaenoic (EPA) and docosahexaenoic (DHA) acids. |