Predators and Parasites
![]() |
Figure 40-10 Classic predator-prey experiment by Russian biologist G. F. Gause in 1934 shows the cyclic interaction between predator (Didinium) and prey (Paramecium) in laboratory culture. When the Didinium find and eat all the Paramecium, the Didinium themselves starve. Gause could keep the two species coexisting only by occasionally i introducing one Didinium and one Paramecium to the culture (arrows); these introductions simulated migration from an outside source. |
The ecological warfare waged by predators against their prey causes coevolution: predators get better at catching prey, and prey get better at escaping predators. This is an evolutionary race that the predator cannot afford to win. If a predator became so efficient that it exterminated its prey, the predator species would become extinct. Because most predators feed on more than a single species, specialization on a single prey to the point of extermination is uncommon.
However, when a predator does rely primarily on a single prey species, both populations tend to fluctuate cyclically. First prey density increases, then that of the predator until prey become scarce. At that point, predators must adjust their population size downward by leaving the area, lowering reproduction, or dying. When density of the predator population falls enough to allow reproduction by prey to outpace mortality from predation, the cycle begins again. Thus, populations of both predators and prey show cycles of abundance, but increases and decreases in predator abundance are slightly delayed relative to those of prey because of the time lag in a predator’s response to changing prey density. We can illustrate this process in the laboratory with protozoa (Figure 40-10). Perhaps the longest documented natural example of a predatorprey cycle is between Canadian populations of snowshoe hares and lynxes (see Figure 30-27).
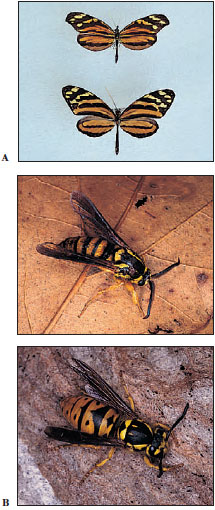
![]() |
Figure 40-11 Artful guises abound in the tropics. A, a palatable butterfly (top photograph) mimics a poisonous butterfly of a different family (lower photograph). B, a harmless clearwing moth (top photograph) mimics a yellowjacket wasp, which is armed with a stinger (lower photograph). Both A and B are examples of Batesian mimicry. C, Two unpalatable tropical butterflies of different families resemble one another, an example of Müllerian mimicry. |
When distasteful prey adopt warning coloration, advantages of deceit arise for palatable prey. Palatable prey can deceive potential predators by mimicking distasteful prey. Coral snakes and monarch butterflies are both brightly colored, noxious prey. Coral snakes have a venomous bite, and monarch butterflies are poisonous because caterpillars store poison (cardiac glycoside) from milkweed they eat. Both species serve as models for other species, called mimics, that do not possess toxins of their own but look like the model species that do (Figure 40-11A and B).
In another form of mimicry, two or more toxic species resemble each other (Figure 40-11C). We can ask why an animal that has its own poison should gain by evolving resemblance to another poisonous animal. The answer is that the predator needs only to experience the toxicity of one species to avoid all similar prey. A predator can learn one warning signal more easily than many!
Sometimes the influence of one population on others is so pervasive that its absence drastically changes the character of the entire community. We call such a population a keystone species.* For example, in 1983, a mysterious epidemic swept through Caribbean populations of the sea urchin Diadema antillarum, destroying more than 95% of the animals. The immediate effect was on the algal community, no longer grazed by the urchins. In some reefs the algae grew from a thin mat to a thick canopy of altered composition. Both productivity and diversity on the coral reefs declined. Diadema antillarum clearly was a keystone predator species for those communities. On the West Coast, the sea star Pisaster ochraceous is a keystone species. Sea stars are a major predator of the mussel Mytilus californianus. When sea stars were removed experimentally from a patch of Washington State coastline, mussels expanded in members, occupying all space previously used by 25 other invertebrate and algal species (Figure 40-12). Keystone predators act by reducing prey populations below the level where resources such as space are limiting. The original notion that all keystone species were predators has been broadened to include any species whose removal causes the extinction of others.
By reducing competition, keystone species allow more species to coexist on the same resource. Consequently they contribute to maintaining diversity in the community. Keystone species can be considered a special case of a more general phenomenon, disturbance. Periodic natural disturbances such as fires and hurricanes also can prevent monopolization of resources and competitive exclusion by a few broadly adapted competitors. Ecologists now believe that disturbances permit more species to coexist in such highly diverse communities as coral reefs and rain forests.
Parasites are often considered freeloaders because they appear to get something from their hosts for nothing.
Ectoparasites such as ticks and lice infect many different kinds of animals. The host provides nutrition from its body and aids in dispersal of the parasite. However, we must consider that the evolutionary pathway to parasitism from free-living forms often has costs as well as benefits. Endoparasites such as tapeworms (see Acoelomate Animals), have lost their ability to choose habitats. Also, because they must move among hosts to complete their life cycle, the chance that a single individual will live to reproduce is very low. The more intermediate hosts involved in a parasite’s life cycle, the lower the likelihood of success, and the greater reproductive output must be to balance mortality.
Biologists often are puzzled by the complexity of parasite-host relationships. For example, one of the trematode parasites of the marine gastropod Ilyanassa obsoleta actually changes its host’s behavior to complete its life cycle. These snails live in sandy-bottomed intertidal habitats in eastern North America. If the snails are exposed to air when the tide recedes, they normally burrow into the sand to avoid desiccation. If, however, a snail is infected with the trematode Gynaecotyla adunca, it moves shoreward on high tides preceding low night tides to be left on the beach on the receding tide. Then, as in the legend of the Trojan Horse, the snail sheds cercariae into the sand where they can infect the next intermediate host, a beach-living crustacean. The crustacean may then be eaten by a gull or other shorebird, the definitive hosts for this trematode. The life cycle is completed when the bird defecates into the water, releasing eggs from which hatch the larvae that will infect more snails.
![]() |
Figure 40-12 The experimental removal of a keystone species, the predatory sea star Pisaster ochraceus, from an intertidal community completely changes the structure of the community. With their principal predator missing, mussels form dense beds by outcompeting and replacing other intertidal species. |
![]() |
A population of giant pogonophoran tubeworms grows in dense profusion near a Galápagos Rift thermal vent, photographed at 2800 m (about 9000 feet) from the deep submersible Alvin. Also visible in the photograph are mussels and crabs. |
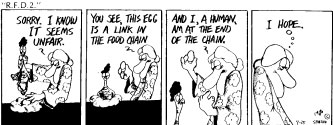
How could this parasite evolve to depend upon the transfer from an aquatic intermediate host to another intermediate host that lives on land? The answer may be that the life cycle of this parasite has been around longer than the crustaceans have occupied land. When the crustacean evolved its terrestrial habit it simply brought the parasite along.
Coevolution between parasite and host may be expected to generate an increasingly benign, less virulent relationship. Selection would favor a benign relationship, because a parasite’s fitness is diminished if its host dies. This traditional view has been challenged in recent years. Virulence is correlated, at least in part, with availability of new hosts. When alternative hosts are common and transmission rates are high, a host’s life is of less value to a parasite, which may become more virulent.