Introduction
Higher plants produce a wide range of chemicals. More than 25,000 terpenoids,
about 12,000 alkaloids, and 8000 phenolic substances have been identified thus far
(Croteau
et al., 2000). These chemicals serve in a variety of functions in plants.
They defend against herbivores and pathogens, aid in interplant competition,
attract beneficial organisms such as pollinators, and have protective effects with
regard to abiotic stresses such as UV exposure, temperature changes, water status,
and mineral nutrients. In addition, many secondary metabolites produced in
plants are used by humans as spices, dyes, fragrances, flavoring agents, or pharmaceuticals.
Many of these chemicals also promote human health and enrich our lives
in many different ways.
The use of plant metabolites as natural medicines has a long history that can be
traced back more than 3500 years, when Egyptian, Sumerian, Ayurvedic, and
Chinese medicines were developed (Askin
et al., 2002). Morphine was isolated
as the principal active ingredient in opium just 200 years ago (1806), and the
pain-killing and fever-reducing aspirin (acetyl salicylic acid) became available via
chemical synthesis, instead of from willow bark extract, only about 100 years ago
(1897). Following rapid progress in chemical synthesis, the microbial production
of antibiotics, as well as biotransformation approaches, has increased the supply
of modern medicines. Today, however, a large proportion (more than 25%) of
products beneficial to humans are still derived from natural sources, especially
from plants (Askin
et al., 2002; Briskin, 2000; Fig. 11.1).
Many medicinal plants are still harvested in the wild due to the technical
difficulties of cultivation, as well as for economic reasons. This harvesting of
medicinal plants along with human disturbance of the natural environment
increasingly raises concerns about diminishing biodiversity. For example,
Taxus
brevifolia (yew), which is used for the production of paclitaxel (Taxol
TM), a potent
antineoplastic agent, is an endangered species on the west coast of the United
States. The increased demand and drastic reduction in plant availability increase
the pressure to produce medicinal compounds in alternative ways, especially via
cell/tissue cultures and transgenic plants since plant cells have a high potential for
totipotency. Furthermore, the demand for quality materials has also increased since variations in medicinal plant quality and incorrect plant identification
occasionally cause tragic consequences. Thus, the application of biotechnological
approaches to produce secondary metabolites is an attractive alternative for their
production, particularly in transgenic cell cultures.
In vitro cell culture systems have several advantages, whose benefits have been
discussed in depth previously (Dougall, 1981). In summary, the desired metabolites
are produced in a controlled environment, independent
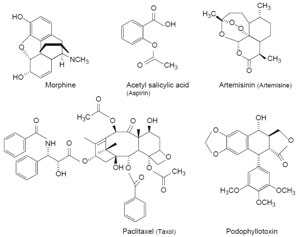 |
FIGURE 11.1 Chemical structures of some
important medicinal compounds. |
of climatic changes
and soil conditions, and free of microbes and insects. In addition, materials of
definitive quality can be produced, and whose productivity can be improved
through cell selection and optimization of culture conditions. The established
cell culture systems are also useful for understanding the synthesis of natural
products since the results obtained are generally uniform and reproducible. The
products are obtained under controlled conditions and are available in large
quantities from cells that are less structurally organized than those of higher
plants. On the other hand, cell culture systems also have several disadvantages;
for example, the labor required to maintain cell culture systems by serial passages,
and the possibility that cell properties may change during serial cultures over long
periods of time or that cultures may be lost due to contamination.
Before 1970, the reported yields from cell cultures were generally lower than
those in plants. However, as shown in Table 11.1, several cell culture systems
can have considerable productivity, and in some cases their production exceeds
than that found in the intact plant. Also some plant cell cultures still produce little,
if any, of the desired compounds. These facts highlight the difficulties faced
in producing useful metabolites in economically viable amounts. Accordingly,
the current advancements in the molecular and cellular biology of secondary
metabolism provide a basis for optimism regarding the commercial production
of secondary products in cell/organ cultures and/or transgenic plants (Facchini
and St-Pierre, 2005; Kutchan, 2005a,b; Zhao
et al., 2005).
Since the biochemistry and cell biology of secondary metabolism represent the
foundation of attempts directed toward biotechnological improvement, we first
review studies that focus on alkaloids for illustrative purposes only. Simplest
applications are biochemical conversions of chemicals that are readily available
using isolated native, or recombinant, enzymes and cells as biocatalysts. More
complicated metabolic engineering attempts require additional biochemical and
cell biological information to optimize the conditions for production. The first
section thus addresses the biochemistry and cell biology and then is directly
related to the application of metabolic engineering.