The Challenge of Lignin Manipulation: Plant Growth/Development Versus Stem Structural Integrity
There are compelling and long-standing reasons to identify novel ways to more
effectively either utilize the lignin biopolymers or manipulate the amounts or
forms of carbon allocated to the lignin-forming pathway, for example, to produce
more desirable bioproducts in commercially cultivated plant species. Indeed,
a number of biotechnological manipulations of both lignin contents and compositions in various plant species have already been carried out; that is,
various transgenic/mutant lines have been successfully obtained using standard
transformation procedures (see Anterola and Lewis, 2002, for examples and
references therein). Generally, though, the effects of drastically reducing lignin
contents in both woody and nonwoody vascular plants result in a significant
impairment/weakening of the vascular apparatus, for example, collapsed vessels
(for a discussion and examples, see Anterola and Lewis, 2002). Such defects
potentially lead to severe drawbacks in growing biotechnologically modified
plant lines commercially, as this can lead to, for example, premature lodging,
weakening of plant stems, and dwarfing during growth/development.
To put the utility of employing mutant and/or genetically modified lines into
sharper focus, the following examples should illustrate why this issue deserves
attention. It is often overlooked that many lignin mutants have been described
over a period spanning nearly a century, particularly the brown midrib mutants
(see Anterola and Lewis, 2002). All had significant
 |
FIGURE 13.5 Effects of knocking out Atcad4
and cad5 (cad-c cad-d) in Arabidopsis thaliana
(ecotype Wassilewskija). (A) Phenotypical
differences between 4-week-old wild type (Ws)
and
lignin-deficient cad-4 cad-5 double mutant
plants. (B) Tensile storage and loss moduli of WT
and
lignin-deficient (~90%) cad-4 cad-5 double
mutant lines. Source: Redrawn from Jourdes et al.
(2007). |
deleterious effects on vascular
tissue integrity, and none, to our knowledge, has yet found commercial application.
Three of these are COMT, CAD, and cinnamoyl CoA oxidoreductase (CCR) mutants (see Fig. 13.1 for biochemical pathway steps). The first, due to introduction
of a 5-hydroxyconiferyl alcohol (
22) monomer in lignin, results in brittle
stems, which are more susceptible to lodging (Anterola and Lewis, 2002). The
CAD double mutation also results in a generally weakened vasculature. The CAD
double mutant in
A. thaliana has a greatly compromised ability to form monolignols
19, 21, and 23 (by ~90–94%), with only very small amounts of lignin proper
being formed (~10% of the natural levels). The resulting stems (of the CAD double
mutant) are thus unable to stand upright (Fig. 13.5A) and their tensile modulus is
greatly compromised (~50%) when tested in the tension mode (Fig. 13.5B)
(Jourdes
et al., 2007). Such a phenotype may be a disadvantage for either largescale
commercial cultivation, harvesting, or processing due to the weakened
vascular apparatus. It is also unknown whether such modifications may also
impact/decrease resistance to opportunistic pathogens.
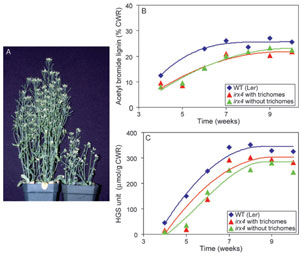 |
FIGURE 13.6 Effects of mutating CCR in
Arabidopsis thaliana. (A) Phenotypical
differences
between wild type (Ler, left) and irx4
mutant (right) plants. (B) Plot of acetyl bromide
lignin
determinations in stems at various stages of
A. thaliana growth and development.
(C) Estimations
of H, G, and S monomer
amounts released during thioacidolysis of wild
type and irx4 extractive-free stem tissues. Source:
Redrawn from Patten et al. (2005). |
CCR mutation in
A. thaliana also resulted in a severely dwarfed phenotype and
a delayed but coherent lignification program (Fig. 13.6A–C) (Laskar
et al., 2006;
Patten
et al., 2005), whereas in tobacco, it resulted in a compromised vasculature and dwarfing as well (Piquemal
et al., 1998). In an analogous manner, 4-coumarate
CoA ligase (4CL), PAL, and C4H downregulation resulted in a significant loss of
vascular integrity (see Anterola and Lewis, 2002) and/or other effects, such as
dwarfing, due (mainly) to reduced lignin levels. Other concerns about deleterious
effects on vascular integrity hold also for C3H downregulation (Patten
et al., 2007).
These examples underscore the central question as to what extent lignin
compositions/contents can actually be manipulated, without introducing structural
defects prohibiting field applications of the resulting plant cultivars in, for
example, bioethanol/biofuel/bioproduct generation. Another possible concern is
that a weaker vascular apparatus may result in plants more susceptible to opportunistic
pathogens. In short, it is becoming increasingly evident that a judicious
balance must be maintained in growing vascular plants for commercial purposes
and in reducing/modifying lignin contents/compositions. However, what flexibility
exists in modifying lignin amounts/composition to avoid such adverse
growth/developmental effects has not yet been determined.